REFERENCE: Sample List of Ion Thrusters
Ion Thrusters for Space Travel
HOWEVER, plasma is a dangerously hot substance which heats up to millions of degrees Celsius; at such extreme heat, plasma can actually generate x-rays. While such heat is unfathomable to most, thruster designers must generate temperatures many times higher. The goal is to create a thruster powered by Nuclear Fusion. Thus, the plasma temperatures must be so extreme the ions actually fuse into larger atoms. The pay-off in terms of energy output is huge. After all, nuclear fusion powers the stars.
EXAMPLE: International Thermonuclear Experimental Reactor (ITER) is a multinational, massive fusion reactor in southern France. For spacecraft ion thrusters, fusion reactors must use same physical process as ITER, but at a much smaller scale.
To convert reactor's energy output into spacecraft thrust, typical engine design will have two layers of plasma:
1) a super-heated core
2) a cooler outer layer.
As hotter ions from the core heat up the cooler plasma, the energy transfer will expand outer layer to push an ion stream out of the aft magnetic nozzle, resulting in thrust. The magnetic nozzle constricts exhaust flow much like a physical nozzle in a conventional rocket.
2) Quantized Inertial (QI)
ELECTRO-THERMAL
Electrothermal thrusters use electromagnetic fields to create plasma to heat the bulk propellant. The thermal energy converts into kinetic energy via a nozzle of either solid material or magnetic fields. Low molecular weight gases (e.g. hydrogen, helium, ammonia) are preferred propellants for this kind of system.
1) Resistojets

Thrust is determined by the resistance heater's capability to rapidly increase temperature and inject heat into the gaseous propellant. Heat energy must then convert into axial kinetic energy as the propellant gas becomes high speed ions to exit aft via exhaust nozzle for forward propulsion. Resisto-jets have been developed since the late 1950's for use on many spacecraft.
2) Microwave Electro-thermal Thruster (MET)
MET uses microwave energy to sustain a plasma in a flowing gas; subsequently, this energy converts into thrust as the ions exit a nozzle. Since microwaves can keep plasma away from the material surfaces of its chamber, MET can greatly reduce erosion from plasma "sputtering" to increase thruster's useful lifetime, compared with more traditional EP thrusters.
MET's microwaves (MWs) tend to reflect back from the plasma; this reflection increases as the gas's temperature and electron density increases. PROBLEM: MET efficiency suffers because reflected MWs don't inject heat to the gas and plasma. SOLUTION: To greatly mitigate this reflection problem, some METs have another microwave cavity for a second plasma cavity in the supersonic part of the MET's outflow.
Neutral propellant injects into an ionization chamber, which might operate on the simple electron bombardment principle (shown in the figure). The gas in the chamber gains some ionization; thus, the ions are extracted due to their charge and mostly ions and electrons exit the chamber.
Expelled ions propel the spacecraft in the opposite direction, per Newton's 3rd law. A separate cathode (neutralizer) emits electrons into the exiting ion beam to neutralize expelled ions. Otherwise, spacecraft would gain a net negative charge to attract ions back toward the spacecraft and cancel the thrust.
At a high potential, cloud's energetic electrons ionize even more neutral atoms in the inert propellant (typically xenon). These positively charged ions (plasma) flow in an electron stream to the exit nozzle to join the high speed, thruster exhaust. As ions exit exhaust, another cathode ("neutralizer") emits electrons to join the positive ions; then, become a quasi-neutral plasma.
HETs, known as "closed drift" thrusters, create an electrostatic field when plasma electrons interact with a magnetic field. HET produces free electrons for two functions:
1) electron flow (current) through the plasma in a longitudinal direction.
2) electron cloud of freely drifting electrons around an anode; this cloud is formed by the "Hall effect".
"Hall effect" comes from free electrons which wind up in orbits perpendicular to the current flow in the plasma to form an electron cloud. Dr. Edwin Hall discovered in 1879 that a properly positioned magnetic field can create an electron cloud around an anode (magnet's positive pole).
Spacecraft leverage the Hall effect for a carefully controlled thrust. Cloud's numerous electrons repulse like charged longitudinal electrons to surge throughout the chamber and collide with propellant atoms. Such collisions create ions to exit the spacecraft to produce sufficient thrust for orbital station keeping operations.
ELECTRO-MAGNETIC THRUSTER
Main Wikipedia article: Plasma propulsion engine If ions are accelerated by the Lorentz force (i.e., due to electromagnetic fields where the electric current direction differs from direction of the ion's acceleration), the device is electromagnetic.
Electromagnetic propulsion (EMP) uses both electrical current and a magnetic field to propel spacecraft. When active current flows through a magnetic field, an electromagnetic force (i.e., Lorentz force) pushes the conductor perpendicular to the magnetic field. This crosswise force can cause propulsion.
In its basic form, the MPD thruster has two metal electrodes:
1) a central rod-shaped cathode,
2) a cylindrical anode around the cathode.
1) A intake nozzle injects a puff of gas across an induction coil.
2) Capacitors release a high voltage pulse for a radial magnetic field.
3) Subsequent electrical field ionizes the gas for a cloud of charged particles (plasma).
4) Ions orbit in the opposite direction as the high voltage pulse.
Because this induced current flows perpendicular to the magnetic field, the plasma accelerates out into space by the Lorentz force at a high exhaust velocity (10 to 100 km/s).
Advantages include:
• While a typical electrostatic ion thruster uses an electric field to accelerate positive ions, a Electro-Magnetic PIT uses the Lorentz body on all charged particles within a quasi-neutral plasma.
• While most other ion thrusters use electrodes, PIT needs no electrodes (which eventually erode)
• PIT power can scale up simply by increasing the number of pulses per second. EXAMPLE: A 1 MW system would pulse 200 times per second.
• PITs can use many gases as a propellant, such as water, hydrazine, ammonia, argon, xenon... EXAMPLE: For Martian missions, a PIT powered orbiter could refuel by scooping CO2 from the atmosphere of Mars, compressing the gas and liquefying it into storage tanks for the return journey or another interplanetary mission, whilst orbiting the planet.
Reverse Field Pinch (RFP)
HOWEVER, some scientists propose thrust from the Quantum Vacuum (QV) acting like propellant ions in a MagnetoHydroDynamics (MHD) drive for spacecraft propulsion. In this model, the MHD ions are replaced by the virtual quantum particles of the QV, eliminating any need for propellant.
This "virtual particle" model was soundly criticized by the scientific community; the QV cannot be ionized and is understood to be “frame-less”; thus, you cannot “push” against it, as required for momentum.
HOWEVER, a NASA sponsored company recently reported success with EM Drive in a hard vacuum; the first known successful EM test. Many scientists now conduct experiments and discuss relevant theories for more knowledge about the EM Drive.
Propellant-less propulsion would drastically reduce fuel resupply requirements such as those needed for International Space Station (ISS) and other long term missions. The elimination of these currently much needed maneuvers could reduce stress on the Station’s structure for a pro-longed ISS operational period as well as future LEO space stations.
Moving out from GEO, experts have noted that a spacecraft equipped with EM drive technology could surpass the performance expectations of the WarpStar-I concept vehicle. A similar vehicle with an EM Drive could travel from the surface of Earth to the surface of the moon within four hours. Such a vehicle could carny two to six passengers and luggage and then return to Earth in another four-hour interval.
Quantized Inertia
Researchers seek light propelled vessel.
Ion thrusters are small, plasma-powered engines on many modern spacecraft. Plasma, collection of ions, can be made in labs by subjecting a gas to a strong electromagnetic field.
![]() |
Variable Specific Impulse Magneto-plasma Rocket (VASIMR) excellent example of a current ion thruster. |
EXAMPLE: International Thermonuclear Experimental Reactor (ITER) is a multinational, massive fusion reactor in southern France. For spacecraft ion thrusters, fusion reactors must use same physical process as ITER, but at a much smaller scale.
To convert reactor's energy output into spacecraft thrust, typical engine design will have two layers of plasma:
1) a super-heated core
2) a cooler outer layer.
As hotter ions from the core heat up the cooler plasma, the energy transfer will expand outer layer to push an ion stream out of the aft magnetic nozzle, resulting in thrust. The magnetic nozzle constricts exhaust flow much like a physical nozzle in a conventional rocket.
![]() |
The main ITER construction site in the south of France |
ELECTRO-THERMAL
1) Resistojets
2) Microwave Electro Thruster (MET)
3) Arcjet
4) DC Discharge Thruster
5) RF Discharge Thruster
ELECTRO-STATIC
1) Gridded Ion Thruster
2) Field Emission Electric Propulsion (FEEP)
3) Colloid Thruster
HALL EFFECT
1) Hall Effect Thruster (HET)
2) HET Types
3) HET Variations
4) Hall Effect Theory
ELECTRO-MAGNETIC
1) Magneto Plasma Dynamics (MPD)
2) Pulsed Inductive Thruster (PIT)
3) Reversed Field Pinch (RFP)
FUTURISTIC CONCEPTS
1) EM Drive1) Resistojets
2) Microwave Electro Thruster (MET)
3) Arcjet
4) DC Discharge Thruster
5) RF Discharge Thruster
ELECTRO-STATIC
1) Gridded Ion Thruster
2) Field Emission Electric Propulsion (FEEP)
3) Colloid Thruster
HALL EFFECT
1) Hall Effect Thruster (HET)
2) HET Types
3) HET Variations
4) Hall Effect Theory
ELECTRO-MAGNETIC
1) Magneto Plasma Dynamics (MPD)
2) Pulsed Inductive Thruster (PIT)
3) Reversed Field Pinch (RFP)
FUTURISTIC CONCEPTS
2) Quantized Inertial (QI)
ELECTRO-THERMAL
Electrothermal thrusters use electromagnetic fields to create plasma to heat the bulk propellant. The thermal energy converts into kinetic energy via a nozzle of either solid material or magnetic fields. Low molecular weight gases (e.g. hydrogen, helium, ammonia) are preferred propellants for this kind of system.
1) Resistojets
Resistance jets (resistojets) energize the propellant via voltage applied to a resistor which heats up accordingly. This increases the Specific Impulse beyond that achievable via traditional chemical reaction. Resistance‐heated thruster (i.e., resistojet) is the simplest form of Electric Propulsion (EP).
Thrust is determined by the resistance heater's capability to rapidly increase temperature and inject heat into the gaseous propellant. Heat energy must then convert into axial kinetic energy as the propellant gas becomes high speed ions to exit aft via exhaust nozzle for forward propulsion. Resisto-jets have been developed since the late 1950's for use on many spacecraft.
![]() |
To reduce MW reflection, METs can add a second plasma cavity. |
MET's microwaves (MWs) tend to reflect back from the plasma; this reflection increases as the gas's temperature and electron density increases. PROBLEM: MET efficiency suffers because reflected MWs don't inject heat to the gas and plasma. SOLUTION: To greatly mitigate this reflection problem, some METs have another microwave cavity for a second plasma cavity in the supersonic part of the MET's outflow.
3) Arcjets
An arcjet thruster creates an electric discharge (arc) in a flow of propellant (typically hydrazine or ammonia). The added energy extracts Specific Impulse out of each propellant particle. While typical arcjet thrust levels are much less than traditional chemical engines, arcjets are much better suited for orbital station keeping.
Researchers have developed various hydrogen-powered arcjet engines capable of power outputs from 1 to 100 kW. The heated hydrogen ions reach exit speeds of 16 km per second (kps).
4) DC Discharge Thrusters
Source Document |
With an applied voltage, cathode accelerates electrons to collide with gas atoms and create ions. The ring-cusp magnetic field (see adjacent figure) keeps both electrons and ions away from chamber surfaces; otherwise:
1) free electrons might "charge" the metal surface to reduce ion speed;
2) free ions (plasma) might impact chamber walls (" sputtering") to eventually damage the spacecraft.
1) free electrons might "charge" the metal surface to reduce ion speed;
2) free ions (plasma) might impact chamber walls (" sputtering") to eventually damage the spacecraft.
The magnetic field guides ions toward the grids which then accelerate ions toward exhaust nozzle. To better understand ion acceleration, consider an exploded view of a single pair of apertures from the screen and accelerator grids.
While traveling toward the screen grid, ions accelerate to max potential of the accelerator grid and then decelerate back to space plasma potential. Discharge chamber electrons bounce off of the screen-grid sheath to reflect back into the chamber. However, other electrons from the neutralizer join the exiting ions to form the beam-ion plasma.
Design variations of the DC ion thruster include:
a) Radio Frequency (RF) design accelerates electrons to ionization energies using power radiated from an antenna, instead of DC power.
b) MicroWave (MW) design uses wave guides to transmit ionization power for the discharge chamber and the neutralizer.
Both variations are simpler than the Direct Current (DC) design because they don't need the hollow cathode in the discharge chamber.
5) RF Discharge Thrusters
Design variations of the DC ion thruster include:
a) Radio Frequency (RF) design accelerates electrons to ionization energies using power radiated from an antenna, instead of DC power.
b) MicroWave (MW) design uses wave guides to transmit ionization power for the discharge chamber and the neutralizer.
Both variations are simpler than the Direct Current (DC) design because they don't need the hollow cathode in the discharge chamber.

Radio Frequency (RF) discharge thruster uses a gas-discharge chamber with an inductor and RF generator. For more, click here.
Another prospective micropropulsion concept uses a capacitive RF discharge between co-axial electrodes. For more, click here.
This RF plasma concept is classified as an electrothermal system. A glow discharge between co-axial electrodes heats a propellant; subsequent thermodynamic expansion generates thrust.
Proof of concept experiments explored electrode wear by comparing DC and RF operation at comparable levels of power, mass flow rate, and electrode separation. The RF mode showed favorable results with respect to surface condition and discharge stability. RF plasma discharges for micro-propulsion devices.
Another prospective micropropulsion concept uses a capacitive RF discharge between co-axial electrodes. For more, click here.
This RF plasma concept is classified as an electrothermal system. A glow discharge between co-axial electrodes heats a propellant; subsequent thermodynamic expansion generates thrust.
Proof of concept experiments explored electrode wear by comparing DC and RF operation at comparable levels of power, mass flow rate, and electrode separation. The RF mode showed favorable results with respect to surface condition and discharge stability. RF plasma discharges for micro-propulsion devices.
ELECTROSTATIC
To avoid excess electric charge on the exit nozzle, a downstream cathode ("neutralizer") injects electrons into ion beam after it exits the nozzle; thus, the gas particles lose their charge to freely disperse in space with no further electrical interaction with the thruster.
If the ion's acceleration is due to the Coulomb force (i.e. application of a static electric field in the direction of the acceleration) the device is electrostatic.

Electrostatic thrusters are also known as "Kaufman" thrusters; they accelerate ions with an electrostatic field. Main elements of an electrostatic thruster are in the following figure.
The gridded ion thruster use high-voltage grid electrodes to accelerate ions with electrostatic forces. Propellant atoms enter the discharge chamber for bombardment by electrons which are typically emitted from a hollow cathode; then, accelerated via potential difference with the anode. These electrons accelerate to higher speeds due to an oscillating electric field induced by an alternating electromagnet.
Then, these high speed electrons collide with propellant atoms to give them a charge. Electrons and charged propellant atoms collectively form plasma, the stuff of stars. The positive propellant particles diffuse towards the chamber's extraction system, two charged grids. After ions enter the plasma sheath via grid holes, they gain even more speed due to the potential difference between the first and second grids (screen and accelerator grids, respectively). Guided through the extraction holes by the powerful electric field, the ion's final energy is determined by the potential of the plasma, generally slightly greater than the voltage of the screen grid.

Small amount of secondary ions escape magnetic containment to erode engine walls to reduce thruster life and efficiency. Since ion engines must run efficiently for years, thruster designers use several techniques to reduce erosion; most notably, use xenon as the propellant, current choice for virtually all ion thruster types. Electrostatic ion thrusters can accelerate ions as fast as 100 km/s.
Conventional electrostatic ion thrusters use a pair of grids; one grid at high voltage and the other low; this single pair does both the ion extraction and acceleration functions. UNFORTUNATELY, some extracted particles inevitably collide with the low voltage grid, eroding it and reducing thruster's engine life. To mitigate this problem, use two grid pairs.
1) First pair operates at high voltage with a high voltage differential (3 kV); this grid pair extracts charged propellant particles from the gas chamber.
2) Second pair, at low voltage, provides the electrical field to accelerate the particles outwards, creating thrust.
This new thruster design has a narrower, less divergent exhaust plume of 3° (previous plume was 15°) to greatly increases precision of thrust vector direction.
Other examples of Gridded ion thrusters include:
1) First pair operates at high voltage with a high voltage differential (3 kV); this grid pair extracts charged propellant particles from the gas chamber.
2) Second pair, at low voltage, provides the electrical field to accelerate the particles outwards, creating thrust.
This new thruster design has a narrower, less divergent exhaust plume of 3° (previous plume was 15°) to greatly increases precision of thrust vector direction.
Other examples of Gridded ion thrusters include:
As an advanced concept for electrostatic space propulsion, FEEP uniquely uses a liquid metal propellant for its ion thruster. FEEP applies a strong voltage (perhaps 10kV) between an emitter and an accelerator electrode. The resultant electric field exacerbates surface tension on the liquid metal (typically caesium, indiumor or mercury) to generate Taylor cones. Eventually, ions emit from these cones via the "electrospraying process"; ions then accelerate to high velocities (typically 100 km/s or more). Due to its very low thrust (from micro-newtons to milli-newtons), FEEP thrusters are best suited for high precision (micro-radians) attitude control of scientific spacecraft.
OPERATIONAL DETAILS: FEEP uniquely provides extremely precise thrusts in the microNewton (μN) to milliNewton (mN) range; this enables accurate thrust modulation in both continuous and pulsed modes. THUS, FEEP has been proposed for attitude control and orbit maintenance on government launched small satellites as well as constellations of miniSats.
Thrust comes from an exhaust beam of mainly singly-ionized liquid metal atoms, produced by electrospray at the emitter tip. Attracting the emitted ions, an accelerating electrode (accelerator) a stainless steel plate contains two sharply machined blades.
To produce exhaust beam, FEEP device applies a high voltage difference between the emitter and the accelerator. Then, the strong electrostatic force changes liquid metal's surface tension to create "Taylor cones"; each Taylor cone "electrosprays" a series of single ions. Collectively, all Taylor cones produce an ion jet to exit exhaust nozzle for a "micro-thrust" to precisely alter vehicle's attitude and/or position.
To produce exhaust beam, FEEP device applies a high voltage difference between the emitter and the accelerator. Then, the strong electrostatic force changes liquid metal's surface tension to create "Taylor cones"; each Taylor cone "electrosprays" a series of single ions. Collectively, all Taylor cones produce an ion jet to exit exhaust nozzle for a "micro-thrust" to precisely alter vehicle's attitude and/or position.
FEEP's Emitter
Typical emitter might have two identical halves of stainless steel, fastened together. A nickel layer, sputter deposited onto one of these emitter halves, outlines the desired channel contour and determines channel height and width (up to about 7 cm).
Colloid Thrusters (NOTE: Colloid Thrusters are essentially same as FEEPs.)
In vivid contrast with the powerful Saturn V rocket, NASA thrusters can also be very precise; their most delicate colloid thrusters max out with same force as the weight of a fine grain of sand.
Precise, delicate, and efficient electrospray thrusters support NASA's Disturbance Reduction System (DRS) as well as nanosatellites. |
Colloids can also last long time; some space borne experiments require 3,000 hours of continuous operational time. Thus, some thrusters use electrospray (also known as "Colloid"), a new technology, which can be both precise and long lasting.
HOW IT WORKS
First, apply an electrostatic field to the surface of an ionized, conductive liquid, perhaps molten salt. Next, the charge distorts the surface to eventually form "Taylor cones". At a certain voltage threshold, a fine spray of ions ejects from the tip of the cone. Finally, these ions electro-statically accelerate to increase thrust.
First, apply an electrostatic field to the surface of an ionized, conductive liquid, perhaps molten salt. Next, the charge distorts the surface to eventually form "Taylor cones". At a certain voltage threshold, a fine spray of ions ejects from the tip of the cone. Finally, these ions electro-statically accelerate to increase thrust.
![]() |
Busek, a commercial firm, developed electrospray thrusters to max out at the weight of a fine grain of sand. |
Busek, Inc. developed a piezoelectric valve to precisely control propellant flow rate. Their electrospray design can efficiently propel nanosatellites, such as the increasingly popular CubeSats, now used by Government agencies, companies, universities, and even hobbyists.
![]() |
Electro-spray thrusters will someday propel nanosatellites like CubeSats. At 10 cm across each edge, CubeSats have tight space constraints. |
Traditional ion thrusters operate at approx. 55% efficiency; thus, about half their input power converts to thrust. They need energy to generate, contain and manage the ionized plasma to propel them. FORTUNATELY, an electrospray system can operate at about 70% efficiency.
Introduction. HET is a plasma propulsion device which can produce low thrust exhausts with great precision for long time. Thus, HET is highly suitable for:
- station keeping,
- orbit transfers,
- interplanetary missions.

HETs, known as "closed drift" thrusters, create an electrostatic field when plasma electrons interact with a magnetic field. HET produces free electrons for two functions:
1) electron flow (current) through the plasma in a longitudinal direction.
2) electron cloud of freely drifting electrons around an anode; this cloud is formed by the "Hall effect".
Spacecraft leverage the Hall effect for a carefully controlled thrust. Cloud's numerous electrons repulse like charged longitudinal electrons to surge throughout the chamber and collide with propellant atoms. Such collisions create ions to exit the spacecraft to produce sufficient thrust for orbital station keeping operations.
![]() |
HET exhaust has distinctive bluish glow. |
How HET Works
The propellant, an inert gas such as xenon or krypton, enters the thruster channel at low pressure. (With inert propellant, HETs avoid explosions, a hazard long associated with chemical rockets.) At one end of HET's plasma chamber, a hollow cathode (negative electrode) generates electrons. At the thruster nozzle, an anode (positive electrode) attracts these electrons. The anode's spike produces a radial magnetic field which collects electrons which spiral around the spike to form a Hall current. During the trip, numerous electrons collide with the propellant atoms to create ions.
HET's electric field moves the positive propellant stream of heavy ions (nuclei of neutrons and protons) through the negative cloud of tiny electrons which accelerates them out of the thruster nozzle in a high speed, ion beam. The thrust is generated by the collective momentum of the high speed ions. Thrust is further enhanced by an external cathode which emits a stream of negative electrons to neutralize the positive ions which might otherwise return to the negatively charged nozzle. 
Two types of Hall Effect Thrusters (HETs) are distinguished by following factors:
1) acceleration zones are different relative lengths.
2) chamber walls are of different materials.
3) discharge plasma uses different physical processes to produce thrust.
ALT's discharge plasma accelerates downstream of the thruster exit plane; thus, the chamber walls suffer no risk from plasma sputtering. Therefore, discharge chamber walls contain a conducting metal with no ceramic protection.
Two types of Hall Effect Thrusters (HETs) are distinguished by following factors:
1) acceleration zones are different relative lengths.
2) chamber walls are of different materials.
3) discharge plasma uses different physical processes to produce thrust.
SPT is a HET type with the acceleration region inside the thruster. Therefore, the chamber walls need a dielectric ceramic material (perhaps boron nitride) as protection from plasma sputtering.
TYPE II: Anode Layer Thrusters (ALTs) ![]() |
The two stages are the ionization stage and the HET used for acceleration. |
To expand HET performance envelope, develop several new thrusters specifically for high Specific Impulse operation. The 2-stage Hall-effect thruster first stage uses an axial magnetic field and antenna for ionization and the second stage uses a traditional HET for acceleration of the ionized particles [Shabshelowitz , 2013].Recent mission studies show the benefits of multi-mode electrostatic thrusters. A multi-mode, or variable Isp thruster, operates at constant power for two operating conditions:
(1) low Isp (1000-2000 sec), high thrust mode suitable for orbit insertion, raising, or repositioning.
(2) high Isp (3000-4000 sec), low thrust mode suitable for station keeping.
2) External Discharge Hall Thruster
Small percentage of plasma ions inevitably escape magnetic containment field to collide with plasma's physical container; at millions of degrees Centigrade, plasma ions will definitely damage any physical object contacted. Over time, continuous sputter erodes discharge channel walls; it also erodes pole pieces which complete the magnetic circuit. Continuing erosion eventually causes thruster to fail. Thus, researchers designed an unconventional Hall thruster design, external discharge Hall thruster or external discharge plasma thruster (XPT). This thruster does not use any discharge channel walls or pole pieces. Plasma discharge is produced and sustained completely in open space outside the thruster structure; thus, we achieve erosion free operation. 3) Magnetically Shielded Hall thruster
Magnetic shielding does reduce discharge channel wall erosion; HOWEVER, pole piece erosion is still a concern. An innovative design called external discharge Hall thruster or external discharge plasma thruster (XPT) does not possess any discharge channel walls or pole pieces. Plasma discharge is produced and sustained completely in open space outside the thruster structure; thus, erosion free operation is achieved.
4) X3 Hall Thruster
The extraordinary X3 Hall design is a significant upgrade from the traditional HET. X3 uses three plasma channels to greatly increase thrust as comparted with the traditional HET with only one channel. X3's current challenge is size reduction; most Hall thrusters can move around a lab with relative ease; however, the much larger X3 needs a crane.
(1) low Isp (1000-2000 sec), high thrust mode suitable for orbit insertion, raising, or repositioning.
(2) high Isp (3000-4000 sec), low thrust mode suitable for station keeping.
2) External Discharge Hall Thruster
Small percentage of plasma ions inevitably escape magnetic containment field to collide with plasma's physical container; at millions of degrees Centigrade, plasma ions will definitely damage any physical object contacted. Over time, continuous sputter erodes discharge channel walls; it also erodes pole pieces which complete the magnetic circuit. Continuing erosion eventually causes thruster to fail. Thus, researchers designed an unconventional Hall thruster design, external discharge Hall thruster or external discharge plasma thruster (XPT). This thruster does not use any discharge channel walls or pole pieces. Plasma discharge is produced and sustained completely in open space outside the thruster structure; thus, we achieve erosion free operation. 3) Magnetically Shielded Hall thruster
4) X3 Hall Thruster
ELECTRO-MAGNETIC THRUSTER
Main Wikipedia article: Plasma propulsion engine If ions are accelerated by the Lorentz force (i.e., due to electromagnetic fields where the electric current direction differs from direction of the ion's acceleration), the device is electromagnetic.
Electromagnetic propulsion (EMP) uses both electrical current and a magnetic field to propel spacecraft. When active current flows through a magnetic field, an electromagnetic force (i.e., Lorentz force) pushes the conductor perpendicular to the magnetic field. This crosswise force can cause propulsion.
EMP can precisely position orbiting satellites. The vehicle's own electromagnetic field can directly interact with the Earth's magnetic field. EXAMPLE: Sat's internal electric current interacts with the natural magnetic field of the Earth; subsequent electro-dynamic force produces thrust. Such EMP systems can provide very precise and instantaneous control over the thrust force. Electrical efficiencies far exceed those of traditional, chemical rockets.
![]() |
Magnetoplasmadynamic (MPD) thruster in operation. Credit: NASA |
Magnetoplasmadynamic (MPD) engine can efficiently convert megawatts of electric power into thrust by expelling plasma ions to propel the vessel. With its high exhaust velocities, MPD propulsion offers distinct advantages over current space propulsion systems.
![]() |
Overview of magnetoplasmadynamic (MPD) thruster operation. Credit: NASA |
In its basic form, the MPD thruster has two metal electrodes:
1) a central rod-shaped cathode,
2) a cylindrical anode around the cathode.
Like an arc welder, a high-current electric arc runs between the anode and cathode. The cathode heats up to emit electrons, which collide with and ionize propellant atoms to create ions, particles of plasma. A magnetic field is created by the electric current completing a circuit back to the cathode, just like the magnetic field that is created when electrical current travels through a wire. This self-induced magnetic field interacts with the electric current flowing from the anode to the cathode (through the plasma) to produce an electromagnetic (Lorentz) force that pushes the plasma out of the engine, creating thrust. An external magnet coil can provide additional magnetic fields to help stabilize and accelerate the plasma discharge.
Pulsed Inductive Thruster (PIT)
A pulsed inductive thruster (PIT) is a plasma propulsion engine using perpendicular electric and magnetic fields to accelerate ions from a propellant; HOWEVER, it has no electrode.
Operation: Following steps:
Operation: Following steps:
2) Capacitors release a high voltage pulse for a radial magnetic field.
3) Subsequent electrical field ionizes the gas for a cloud of charged particles (plasma).
4) Ions orbit in the opposite direction as the high voltage pulse.
Because this induced current flows perpendicular to the magnetic field, the plasma accelerates out into space by the Lorentz force at a high exhaust velocity (10 to 100 km/s).
Advantages include:
• While a typical electrostatic ion thruster uses an electric field to accelerate positive ions, a Electro-Magnetic PIT uses the Lorentz body on all charged particles within a quasi-neutral plasma.
• While most other ion thrusters use electrodes, PIT needs no electrodes (which eventually erode)
• PIT power can scale up simply by increasing the number of pulses per second. EXAMPLE: A 1 MW system would pulse 200 times per second.
• PITs can use many gases as a propellant, such as water, hydrazine, ammonia, argon, xenon... EXAMPLE: For Martian missions, a PIT powered orbiter could refuel by scooping CO2 from the atmosphere of Mars, compressing the gas and liquefying it into storage tanks for the return journey or another interplanetary mission, whilst orbiting the planet.
Reverse Field Pinch (RFP)
A reversed-field pinch (RFP device can produce and contain near-thermonuclear plasmas. RFP uses a toroidal pinch (unique magnetic field configuration) to magnetically encase a plasma. Its magnetic geometry differs from the more common tokamak. As one moves out radially from the center, the toroidal portion of the magnetic field reverses its direction, thus, "reversed field".
Compared to the Tokamak (magnetic strengths in toroidal directions exceeds mag strength of poloidal),
1) RFP's toroidal mag field strength is very near poloidal
2) RFP's toroidal field flows in opposite direction as tokamak.
3) Typical RFP magnetic field strength is much less (about 10% to 50%) than a comparable Tokamak.
4) RFP leverages plasma's driving current to reinforce the magnetic field.
Compared to the Tokamak (magnetic strengths in toroidal directions exceeds mag strength of poloidal),
1) RFP's toroidal mag field strength is very near poloidal
2) RFP's toroidal field flows in opposite direction as tokamak.
3) Typical RFP magnetic field strength is much less (about 10% to 50%) than a comparable Tokamak.
4) RFP leverages plasma's driving current to reinforce the magnetic field.
Electromagnetic microwave cavities might directly convert electrical energy to thrust without the need to expel any propellant. Traditional researchers were initially very skeptical; with no expelled particles, EM Drive seems to violate the law of momentum conservation where spacecraft's forward progress equals combined thrust of exhaust particles.
SEE ALSO |
---|
This "virtual particle" model was soundly criticized by the scientific community; the QV cannot be ionized and is understood to be “frame-less”; thus, you cannot “push” against it, as required for momentum.
HOWEVER, a NASA sponsored company recently reported success with EM Drive in a hard vacuum; the first known successful EM test. Many scientists now conduct experiments and discuss relevant theories for more knowledge about the EM Drive.

FURTHERMORE, EM drive technology could enhance Geostationary Equatorial Orbit (GEO) satellite missions around Earth. For a typical GEO communications satellite with a 6kW (kilowatt) solar power capacity, replacing the conventional apogee engine, attitude thrusters, and propellant volume with an EM Drive could reduce launch mass from 3 tons to 1.3 tons. The satellite could initially launch into Low Earth Orbit (LEO), to deploy its solar arrays and antennas. The EM-drive could then propel the satellite in a spiral trajectory up to GEO in 36 days.

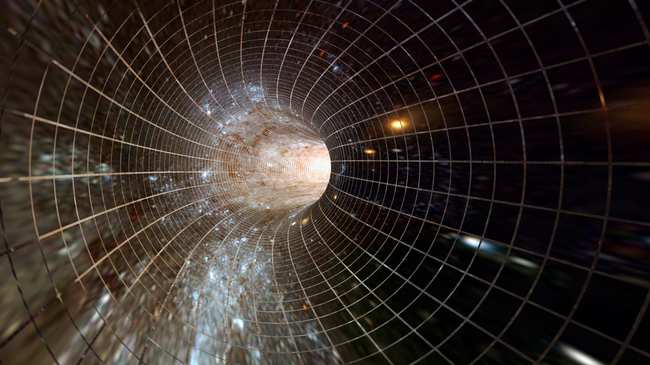
Quantized Inertia (QI), an alternative theory of inertia, might one day propel space vehicles without fuel. An international research team will conduct a series of experiments in a specially equipped laboratory. They will create experimental QI engines with incredibly sensitive detectors. Someday, this work might lead to thrust for satellites for stable, precise orbits; maybe even interstellar travel and other “impossible” applications.
BACKGROUND: An object’s inertial mass is the object’s resistance to acceleration. Imagine punching a glass of water versus punching a fire hydrant with same force, the less massive object (glass of water) will experience much more acceleration than the more massive hydrant.
Inertial mass contrasts with another way to define mass, gravitational mass, the object’s ability to attract other objects. In modern physics, every relevant experiment confirms an object’s inertial mass to equal its gravitational mass. This relationship was stated by Albert Einstein in the early 20th century as the “equivalence principle,” observer cannot distinguish between the effects of a constant gravitational field and a constant acceleration. This was Einstein's starting point for his Theory of General Relativity. Yet in the 20th century, it became increasingly difficult to reconcile this principle with cosmological observations.
BACKGROUND: An object’s inertial mass is the object’s resistance to acceleration. Imagine punching a glass of water versus punching a fire hydrant with same force, the less massive object (glass of water) will experience much more acceleration than the more massive hydrant.
Inertial mass contrasts with another way to define mass, gravitational mass, the object’s ability to attract other objects. In modern physics, every relevant experiment confirms an object’s inertial mass to equal its gravitational mass. This relationship was stated by Albert Einstein in the early 20th century as the “equivalence principle,” observer cannot distinguish between the effects of a constant gravitational field and a constant acceleration. This was Einstein's starting point for his Theory of General Relativity. Yet in the 20th century, it became increasingly difficult to reconcile this principle with cosmological observations.
Slower Star Rotation per conventional physics. | Faster Star Rotation observed by Rubin and others. |
---|
Per conventional physics, the force of gravity decreases with distance; thus, stars should rotate around their galactic center in slower speeds as their radius increases. HOWEVER, observed rotation of individual member stars is much quicker. In the 1970's, Vera Rubin’s galactic observations demonstrated that rotational velocities of stars remain the same, regardless of radial distance from their galactic center. Since then, numerous observations of individual stars in several galaxies have further confirmed this. To explain this phenomena, many scientists now propose "Dark Matter" as a popular theory to explain this discrepancy.
HOWEVER, some scientists instead propose Quantized Inertia (QI) as an alternate theory to Dark Matter. By decoupling an object’s inertial mass from its gravitational mass, QI might explain the observed galactic rotations using only their visible matter.
Unruh Effect. An object's inertial mass is perhaps better explained by Unruh radiation on the object. The Unruh effect states that an accelerating object will perceive an increase in temperature (due to Unruh radiation) not noticed at a constant speed.
HOWEVER, some scientists instead propose Quantized Inertia (QI) as an alternate theory to Dark Matter. By decoupling an object’s inertial mass from its gravitational mass, QI might explain the observed galactic rotations using only their visible matter.
Unruh Effect. An object's inertial mass is perhaps better explained by Unruh radiation on the object. The Unruh effect states that an accelerating object will perceive an increase in temperature (due to Unruh radiation) not noticed at a constant speed.
![]() |
Physics from the Edge Unsure whether the Unruh effect has ever been observed. |
A dark matter alternative might create a light powered propulsion system. A “shield” might dampen the Unruh radiation produced by a highly accelerated object to increase the object’s acceleration.
Perhaps, it could convert light into thrust as well as enhance current thrust systems. Space travel could gain huge benefits from QI propulsion. Current rockets requires large amounts of chemical propellant to reach escape velocity. Larger payloads require more fuel, and more fuel, itself, increases rocket mass which needs yet more more fuel, and so on.
HOWEVER, a QI-based thruster only needs enough power to fuel an electric core. With sufficient acceleration, this core might produce enough Unruh radiation to propel the craft. A research team is now investigating this concept.
Perhaps, it could convert light into thrust as well as enhance current thrust systems. Space travel could gain huge benefits from QI propulsion. Current rockets requires large amounts of chemical propellant to reach escape velocity. Larger payloads require more fuel, and more fuel, itself, increases rocket mass which needs yet more more fuel, and so on.
HOWEVER, a QI-based thruster only needs enough power to fuel an electric core. With sufficient acceleration, this core might produce enough Unruh radiation to propel the craft. A research team is now investigating this concept.
0 Comments:
Post a Comment
<< Home